The rapid expansion of global oil and gas exploration from the early 1960s was accompanied by the expansion of the areas being examined by explorationists towards more challenging, often frontier, plays with the concomitant risks associated.
In the North Sea, for example, early success resulted from standard approaches in the Rotliegendes and Chalk plays that were comparable to onshore successions. However, the discoveries of the Montrose, Forties and Ninian fields resulted from the efforts of the highly skilled and pioneering exploration geologists utilising what were then new approaches to stratigraphic modelling and exploration.
The main stratigraphic techniques employed during the 1960s were lithostratigraphy, wireline logging and biostratigraphy, which, when combined with 2D seismic data, provided the foundation for the stratigraphic frameworks on which most stratigraphic correlations were based. In some circumstances however, these tools alone were unable to produce reliable field- and reservoir-scale correlations, particularly with respect to the predominant sandstone architectures found in the Triassic, Devonian, Upper Carboniferous and Rotliegendes successions in the North Sea. Operators began to look to alternative stratigraphic and correlations tools that could be successfully applied to these successions. Extensive pilot studies were undertaken using various techniques, including magnetic stratigraphy, heavy mineral stratigraphy, clay mineral stratigraphy and whole rock geochemistry incorporating elemental chemostratigraphy.
First Large-Scale Applications of Chemostratigraphy
The potential for characterising sedimentary rock successions using geochemistry has long been known and the significance of the stratigraphic variations in rock geochemistry was already a mainstay in hydrocarbon exploration, using the gamma ray tool and interpreting the resultant logs. Even so, a much more refined approach was required in order to differentiate and correlate lithologically-repetitive strata. At the time, Wavelength Dispersive X-ray Fluorescence (XRF) utilising fused discs and pressed pellets was the chief method for acquiring quantitative data for elements. Large sets of accurate data were obtained, but the process proved to be slow and expensive and depended on relatively large volumes (~4 gm) of sample material being available for analysis. Alternative analytical approaches such as atomic absorption and neutron activation proved likewise sub-optimal for the large-scale, multi-element analytical programmes required by the petroleum industry.
The advent of inductively-coupled plasma (ICP) technology, used with optical emission
spectrometry and mass spectrometry, transformed elemental analysis. These techniques enabled accurate and precise simultaneous measurements of over 50 elements from tiny sample sizes. One of the earliest full-scale deployments of this technology for chemostratigraphic purposes was in 1991 by Tim Pearce (Chemostrat’s CEO International), who applied the technique to Quaternary sediments in the Atlantic, drawing on innovative research conducted by Dr Ian Jarvis and colleagues in the late 1980s. This led to the first large-scale application of chemostratigraphy to the offshore North Sea Triassic sequences in 1992 for Phillips Petroleum.
The success of this application resulted in the technique being adopted by Conoco, Shell and ARCO to help correlate the Upper Carboniferous Barren Red Measures in the North Sea Southern Gas Basin. Detailed interpretation of the elemental data led to the stratigraphy of these formations being refined on both a reservoir- and field-scale and it soon became clear that chemostratigraphy was a valuable and proven addition to the stratigraphic workflow in the context of barren successions or those with poor biostratigraphic control.
Chemostratigraphic data and interpretations complemented existing biostratigraphic schemes and in doing so, effectively initiated the first multidisciplinary approaches to stratigraphy, and during the 1990s chemostratigraphy became adopted by enthusiastic advocates within several oil companies.
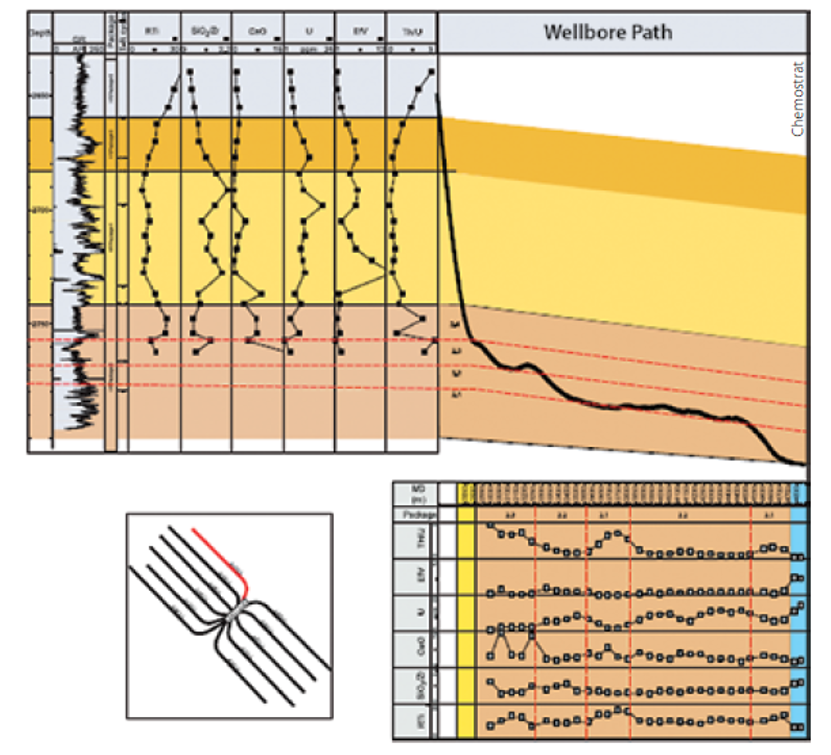
Despite the success of chemostratigraphy during the 1990s, the technique remained a little known, and even less understood, niche application, the subject of active and vociferous debate between its supporters and detractors. Stratigraphy remained synonymous with biostratigraphy, while geochemistry remained synonymous with organic geochemistry for many years.
Choose Carefully
The recent significant rise in the deployment of chemostratigraphy in global exploration largely reflects more rigorous approaches to the generation of geochemical data. Much previous work was hampered by poor understanding of sample quality management, instrument calibration and the critical importance of selecting the correct analytical instruments for the particular situation. Geochemical data can be obtained from sedimentary rocks in several ways and each analytical method can produce accurate and significant data, but the ultimate suitability of any analytical technique is governed by the technical and commercial objectives of the study in question. Focus on particular analytical techniques often results in solutions being deployed that are inappropriate because of a lack of sensitivity of the analytical instruments, or the use of instruments that are not calibrated or monitored correctly for the range of geochemical compositions encountered over the successions being analysed. Understanding the geological setting and basin development, plus the depositional and sedimentological architecture development on a regional basis, is of huge importance in selecting the appropriate analytical tool on which to carry out a chemostratigraphic workflow.
Not all geochemical data currently reported benefit from the care needed in selecting carefully the appropriate technical workflow to analyse cuttings samples in particular, or provide confidence in the reliability and quality of the data. Programmes run by the International Association of Geoanalysts review data to ensure that claims of accuracy and suitability of specific systems are benchmarked, helping explorationists to understand when particular techniques are suitable, and analysts to avoid having to defend specific techniques against less expensive, and sometimes much less suitable, data.
The need for high quality data to be acquired quickly and cheaply has created a drive to develop additional portable and scanning tools. Each technique has its own role to play, but the choice as to which one to use needs to be made with advice; cases abound of organisations buying equipment and attempting to use it in-house, before finding they lacked the requisite experience in analysis and chemostratigraphy, and possibly dismissing the technology.
By carefully selecting the appropriate tools, considering the questions requiring an answer, and bearing in mind budget constraints, it is possible to apply the range of analytical workflows best suited to the problem at hand. In this way chemostratigraphy been rejuvenated within the industry and is applied now throughout conventional and unconventional exploration.
An important point to understand is that chemostratigraphy is not the analytical technique or the instrument used; it is the skilled and experienced interpretation of the data generated and the knowledge of what can and cannot be done with each potential analytical tool. It is the role of chemostratigraphers to showcase to the petroleum industry the immense value of these techniques, which can be very powerful indeed, when carried out with care and skill and combined with geological knowledge. Perhaps the most important challenges faced are created by the fallacious correlation of chemostratigraphy with XRF or ICP or any of the other techniques. An analytical tool generates chemical data, not chemostratigraphy.
Uses of Chemostratigraphy
Chemostratigraphy is especially useful when used in conjunction with other stratigraphic techniques. In most cases, chemostratigraphy is not tied to chronostratigraphy and therefore should be considered as a rock characterisation tool that can be linked to sequence stratigraphy, palaeoclimate and provenance when employed on a regional scale, with the chemostratigraphic interpretations being constrained by either biostratigraphic or isotopic chronostratigraphic frameworks.
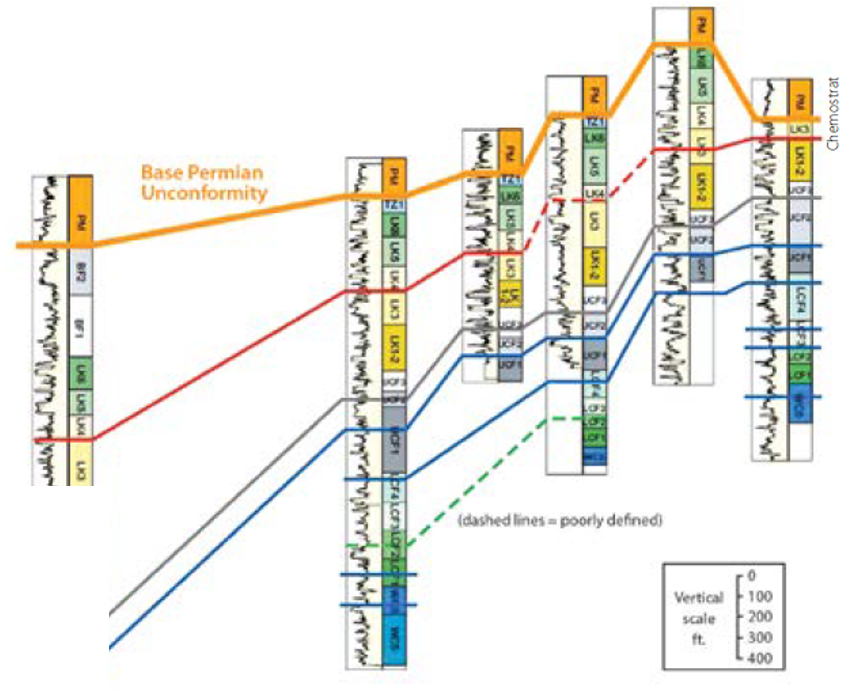
Throughout the 2000s, laboratory-based chemostratigraphic services were routinely applied to carbonate plays, particularly across the Middle East, focusing on providing a geochemical characterisation of the carbonate successions that had subbiostratigraphic resolution. The technique was also employed to correlate ‘tight’ sandstones in North Africa and the Middle East, where interpretation of geochemical data provided insights into facies differentiation, stratigraphic and lateral variations in grain size and mineralogy and possible controls on reservoir quality. The application of chemostratigraphy continues to provide critical information to explorationists as the understanding of its relationship with other techniques is better demonstrated and understood. In conventional exploration, chemostratigraphy is now applied from early wellsite through to production and EOR phases of the oil cycle, with the initial datasets providing key information to geologists, engineers, geophysicists and other disciplines.
The concept of wellsite chemostratigraphy (not to be confused with wellsite XRF) was developed at Chemostrat back in the late 1990s and is now widely applied as a backup option to other wellsite techniques and as a drilling control tool in its own right. This obviously provided the experience and knowledge to apply the technique to the growing shale revolution in the USA in particular. Of course, the shale revolution has provided an entirely new set of defining requirements for geochemical techniques, and chemostratigraphy has proved as robustly valuable in this sphere as it has elsewhere.
When shale exploration first started, chemostratigraphy was routinely used as a correlation tool within a multi-disciplinary approach to stratigraphy. However, once such drilling entered the production stage, the requirement for quantitative data relating to 50 elements diminished. It became clear that rock mechanics needed to be determined more accurately along the length of the lateral boreholes. Traditionally, the analysis of cuttings would involve a lengthy laboratory-based analytical programme, but Chemostrat and others developed bespoke, near real-time analytical procedures that provide an elemental, mineralogical and total organic content data package entirely fit for purpose in this different and challenging sphere of exploration. The data acquired relates to the major elements and some trace elements; these data have moderate analytical resolution compared to data obtained via laboratory-based ICP analyses, but their accuracy is constantly monitored throughout a drilling campaign to ensure they are of sufficient quality to map reservoir brittleness across a field and to constrain a targeted completion programme.
Opportunities Abound
The last oil price decline forced companies to seek increasingly high value for money throughout their workflows, with chemostratigraphy similarly needing to demonstrate a more flexible approach. Prior to the crash, typical pilot deployments of the technique would include five or six wells for international clients, and a significant application could run over many wells and take up to three years. These sorts of approaches have faded, and in some ways explorationists have dismissed the geological workflow in all but select cases. However, the revolutionary approach developed for shale successions will transform the workflows for frontier tight rock and traditional conventional fields alike and will provide additional boosts to ongoing enhanced oil recovery technology. These changes should be recognised as opportunities to deploy more innovative and robust, faster, cheaper technologies and solutions to provide the new drivers of the industry with critical data.