Microfossils are those fossils that require a microscope to carry out fundamental identification. They are the fossils of a biologically diverse group of organisms, including microscopic algae, protists, and invertebrates, and are noted for their geological utility.
Many groups of microfossils exhibit a rapid evolution rate as a result of sensitivity to palaeoenvironmental conditions. As a consequence, they often include short-lived species that have excellent value in calibrating age, correlation and assessing palaeoenvironmental conditions.
Microfossils are therefore essential to biostratigraphy and for several intervals of geological time they are the diagnostic zone fossils. Microfossils are abundant in most sedimentary rocks, and because of their small size are more likely to be preserved and recovered from well cuttings and core than macrofossils are (see also: GeoExpro 2022-5, 72-75).
They provide a basis for subsurface correlation, geochemical studies, understanding of palaeoenvironments, and even source rock maturity. Under the right palaeoceanographic conditions, microfossils have also been deposited in such vast numbers that they are the primary material for the accumulation of some extremely economically important types of rock, including chalk and siliceous rocks such as chert and porcelanite.
Varied Habitats
Microfossils include some groups of organisms which remain extant today (for example dinoflagellates, ostracods (Figure 1) and foraminifera) and others that are now extinct (for example chitinozoans and conodonts). Extant taxa are particularly useful, as a sound understanding of their biology and ecological niches can be used to drive palaeoenvironmental interpretation and reconstruction from fossil occurrences.
Microfossils represent a very diverse suite of zoological and botanical groups of organisms from single-celled to multicellular organisms and larger animals that we only find fragmentary remains of (e.g. conodonts). Like macrofossils, microfossils represent flora and fauna from varied habitats, from marine to terrestrial environments, and across the full aquatic realm from the photic zone to the benthos on the sea floor and across all water depths.
Ostracods, such as the example in Figure 1, are the fossils of tiny crustaceans (arthropods). They are remarkably well adapted to aquatic environments including a variety of marine, brackish and freshwater habitats and can even survive on land provided there is sufficient moisture.
By contrast, the foraminifera (“forams”) are the fossil remains of protists that represent a component of both the marine zooplankton (Globigerinina suborder) and the benthos (11 suborders including the agglutinated forams from the suborder Textulariina). Benthic forms adapted to live on, and in, the top layer of the seafloor substrate and are very sensitive to seawater temperature, salinity and other environmental conditions. This makes them excellent indicators for palaeoenvironmental reconstruction. Care is needed in foram identification – in the Globigerinina alone, of the 2000+ described planktonic foraminifera as many as half probably represent synonyms of already described species.
Other groups like the acritarchs include more than 10,000 described fossils that are of poorly resolved affinity. Then there are the radiolaria, the calpionellids, the chitinozoans, dinoflagellates, silicoflagellates, diatoms, palynomorphs (spores and pollen) and many more. It is impossible to cover everything in this short article, so if you fancy a bit of further reading check out Howard Armstrong and Martin Brasier’s classic textbook “Microfossils” or Marius Dan Georgescu’s “Microfossils through time: an introduction.”
Giant Forams for Giant Fields
Some foraminifera grew so big that they could be regarded as macrofossils (Figure 2). They are an important component in the formation of nummulitic limestones which take their name from the Nummulites foraminifera that are the dominant bioclastic grains in those rocks. One such accumulation of nummulitic limestones is the Eocene (Ypresian) El Garia Formation, a prolific hydrocarbon play along the North African margin.
Numerous discoveries have been made in these limestones offshore Tunisia (including the Ashtart and Zarat fields amongst others), and in offshore Libya where the Giant Bouri field in concession NC-41 is the largest producing oil field in the Mediterranean Sea. In Bouri, banks of Nummulites foraminifera were accumulated as a result of hydrodynamic reworking and comprise the primary reservoir facies in the field.
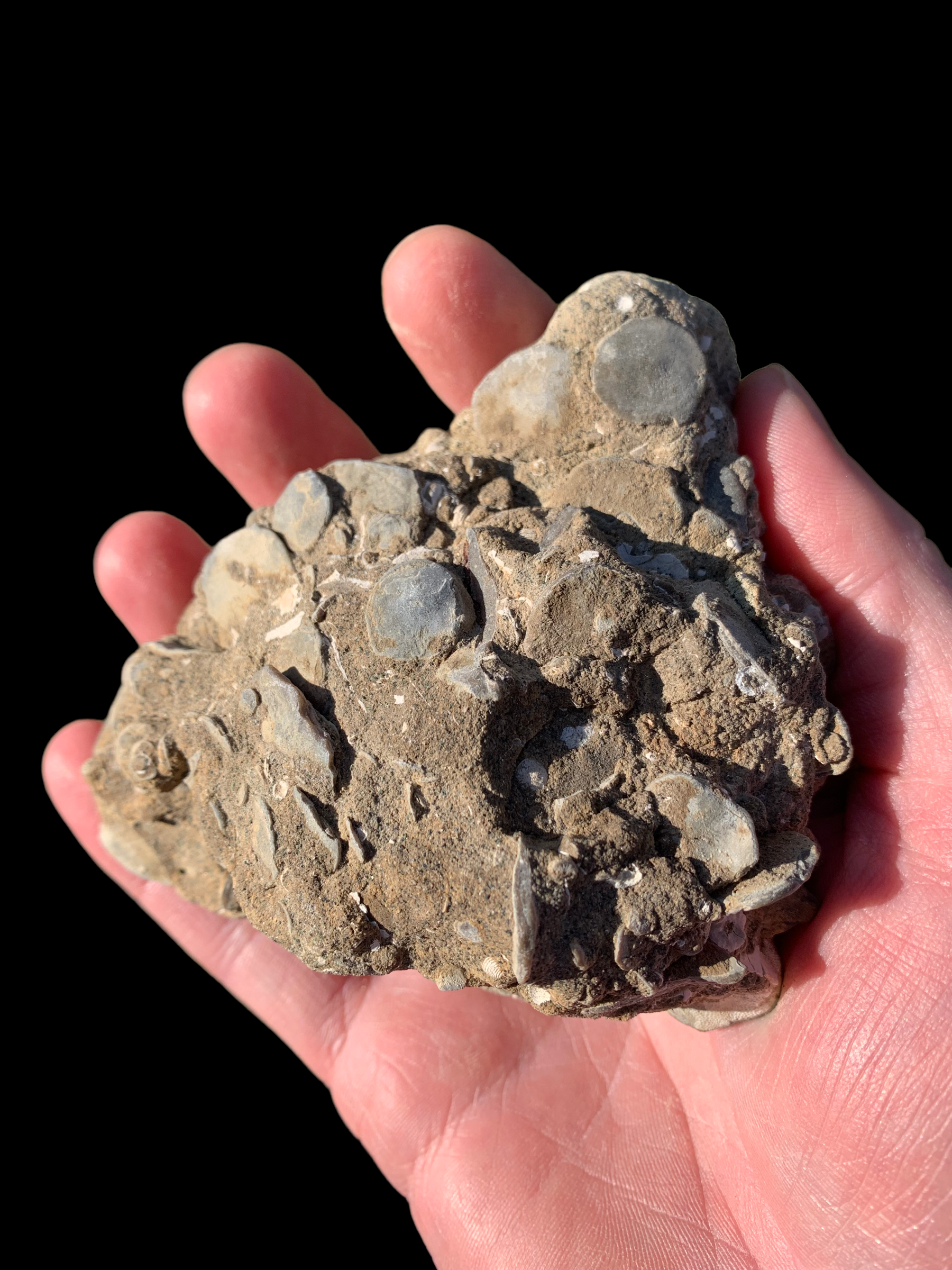
Chalk and Ekofisk
Last year, the Ekofisk field (Figure 3) in the Norwegian sector of the North Sea celebrated 50 years and over 3 billion barrels of cumulative oil production. The field was discovered in 1969 by Phillips Petroleum Company and produces from a 300 m thick oil column 3 km below the modern sea bed in Chalk reservoirs of the Paleocene Ekofisk Formation and the late Campanian to Maastrichtian Tor Formation. Both formations vary in thickness up to 150 m across the Ekofisk area. The Cretaceous Chalk is regionally extensive (Figure 4) and can reach thicknesses of up to 400 m.
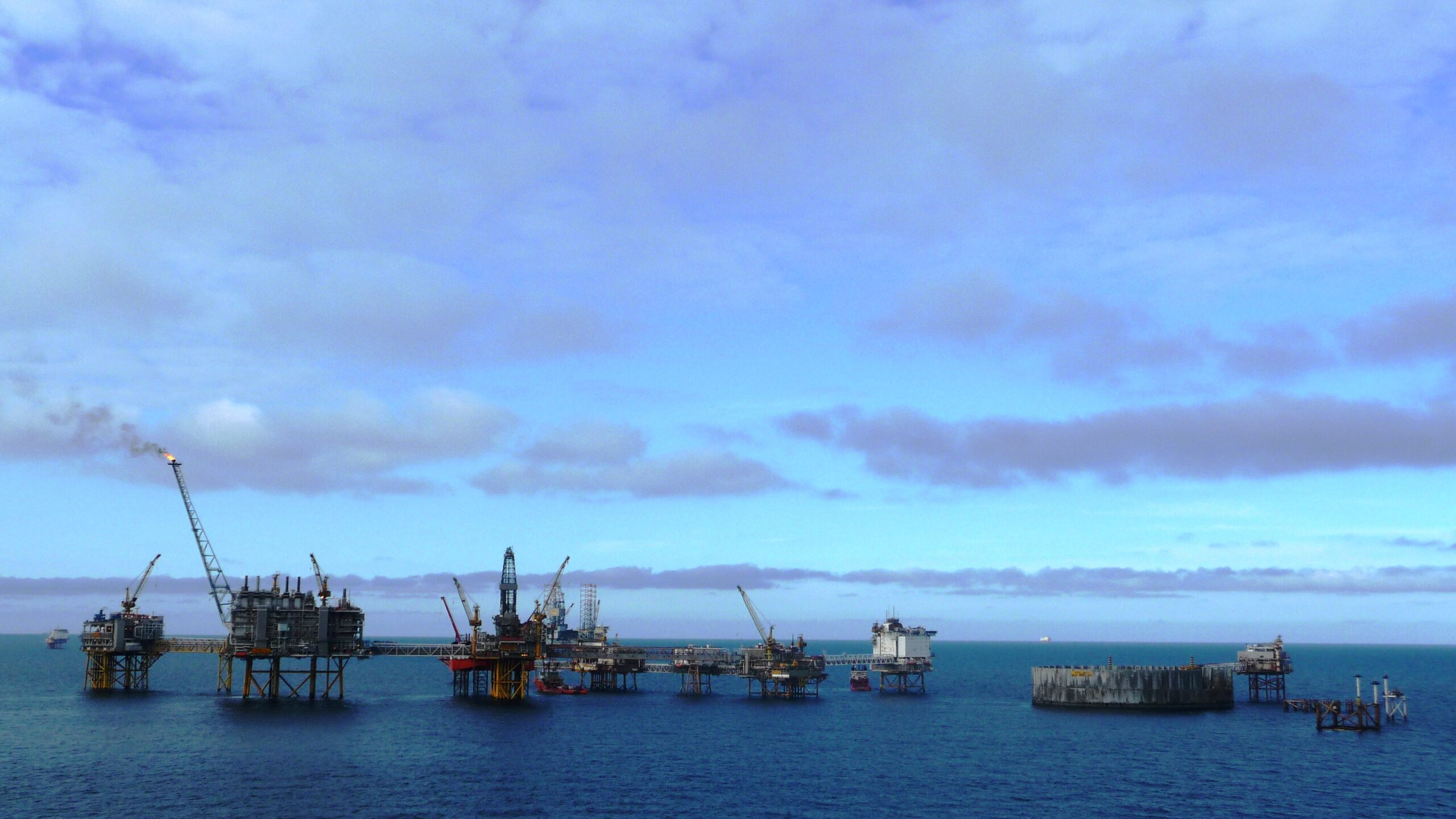
Chalk is essentially a fine-grained limestone composed dominantly of the calcified skeletal debris of haptophyte algae called coccolithophores (Figure 5). Other microfossil grains include planktonic foraminifera and sponge spicules and other minor components (debris from echinoderms for example).
In the Ekofisk area, reservoir properties are excellent with 30-48% interparticle microporosity. Up close, there is a surprising amount of stratigraphic and facies variability in Chalk successions, but from a reservoir perspective the critical factors are pore throat size and tortuosity. Permeability is tight at 1-5 mD but is significantly enhanced by natural fractures (up to 100 mD in places).
Fracture porosity does not contribute much in terms of pore volume but greatly enhances horizontal and vertical permeabilities. A significant body of literature exists on the Chalk with extensive case studies available from numerous fields. It is clear that reservoir quality is impacted by the original depositional facies, burial diagenesis and the timing of oil migration and saturation.
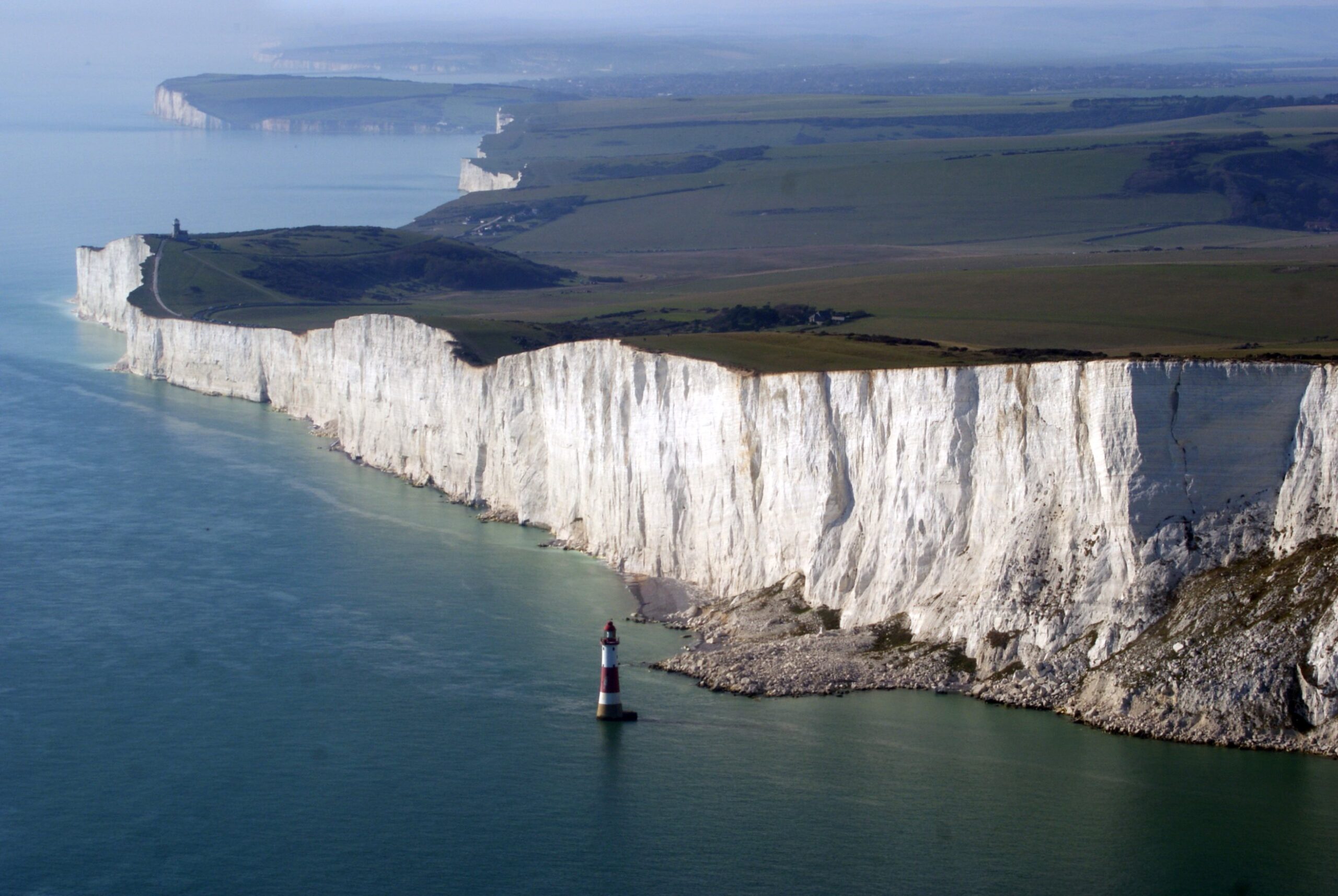
Ekofisk was a ground-breaking discovery and highlighted the significance of the Chalk as an important hydrocarbon reservoir in the North Sea – tens of billions of barrels of oil-equivalent hydrocarbon resources in place have subsequently been identified in North Sea Chalk reservoirs, and with modern stimulation and enhanced oil recovery methods will continue to provide important production.
Optimised well placement in Chalk reservoirs has been facilitated by biosteering using detailed zonation schemes for microfossil assemblages. Some of the higher porosity zones in the Ekofisk Formation are related to debrites which include reworked Cretaceous microfossils mixed with in situ Danian (Paleocene) taxa. The proportions of reworked taxa versus those in situ are sufficiently diagnostic to identify which debris flow unit is being drilled and ensure wells are landed and maintained in-zone to maximise reservoir contact. This approach has kept many Chalk fields profitable beyond original expectations.
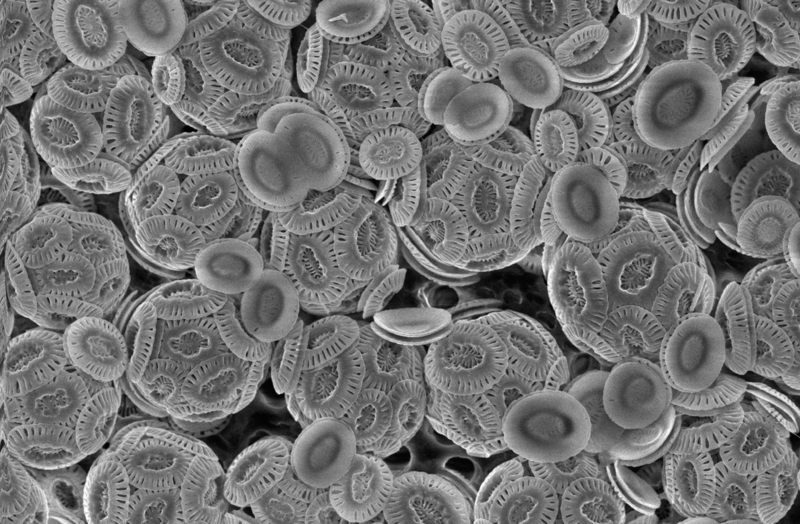
Palaeoclimatology
Because of their sensitivity to palaeoenvironmental conditions, many groups of microfossils are incredibly useful as palaeoclimatic indicators. Palynomorph assemblages are important indicators in terrestrial environments where floral assemblages react very quickly to changes in temperature and aridity. Foraminifera, along with ostracods are arguably the most important groups for such analysis in the marine realm, with specific indicator species, certain morphotypes, composition of microfossil assemblages and preservation styles all relevant to deducing palaeoenvironmental conditions. A number of statistical techniques are used to assist in the analysis of assemblage data.
Foraminifera can provide proxy data for water depth, temperature, salinity, bulk water chemistry, pH, degree of mixing, oxygenation levels of bottom waters and more. These trends can also be related to broader changes in eustacy, oceanic circulation and the opening and closure of oceanic gateways resulting from geodynamic events. Under the right conditions, extremely high rates of organic matter burial can even perturb the carbon cycle.
Diatomites, Chert and Porcelanite
One such event is thought to have occurred during the middle to late Miocene where a change in thermohaline circulation drove the intensification of upwelling around the Pacific Rim, reflected by the widespread deposition of diatomites. Diatoms are photosynthesising green algae that have a siliceous frustule or skeleton and are the primary producers in the world’s oceans today. Diatomite sedimentation was broadly coincident with significant cooling and expansion of the West Antarctic ice sheet and a positive carbon isotope excursion reflecting rapid global drawdown of carbon.
At least some contribution to this event is thought to be associated with the deposition of the Monterey Formation in California. The Miocene Monterey Formation is a thick and organic-rich succession of rocks including mudstones, dolomites, limestones, diatomites, chert and porcelanite. These rocks are the source of most of the conventional oil in California (nearly 40 billion barrels), a succession that is particularly rich in biogenic silica derived from diatoms, silicoflagellates and radiolaria.
In the Monterey Formation, diagenesis of biogenic silica (Opal A) has led to both Opal CT and stable diagenetic quartz cementation, which vary depending on temperature as a function of burial depth. Some fields produce directly from these chert and porcelanite beds, including two reservoirs in the Elk Hills field (onshore) which have delivered cumulative production of more than 80 million barrels from laminated porcelanites with quartz-phase mineralogy. The rocks are tight (0.8 mD) but are enhanced by natural fractures. Elsewhere, in the offshore, production from the Monterey comes from matrix porosity associated with rocks in the Opal CT phase (also enhanced by natural fractures, e.g. Figure 6).
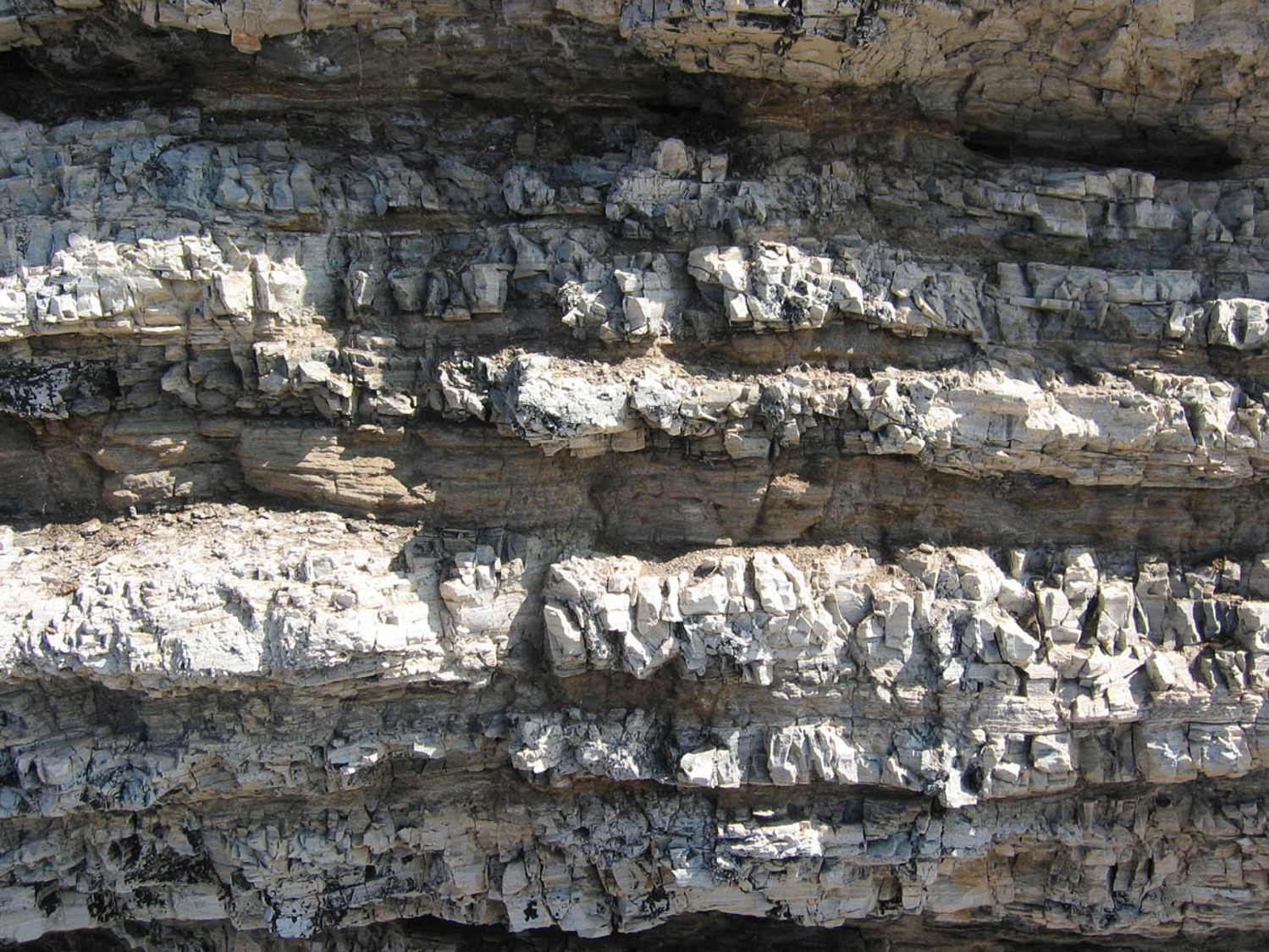
In the Monterey, as with Chalk reservoirs, the timing of hydrocarbon generation and reservoir saturation also plays a role in how porosity is distributed in the resulting diagenetic cements. Natural fractures are known to enhance reservoir characteristics for these otherwise tight rocks, but with additional structural complexity this is not a simple unconventional resource target. Understanding the role microfossils play in the formation and diagenesis of these rocks has been key to understanding reservoir characterisation – a lesson learned as the Monterey is applied as an analogue to siliceous reservoirs elsewhere in the world.
From Luca to Lucy
In summary, microfossils represent a remarkably diverse suite of zoological and palaeobotanical groups. They are incredibly important for subsurface interpretation, facilitating correlation, palaeoenvironmental interpretation, thermal maturity assessments and many other industrially and academically important uses. They have also led to the generation and accumulation of significant volumes of hydrocarbons, and will no doubt influence our understanding of the storage potential (and challenges) associated with depleted fields and saline aquifers for CO2 storage.
This article wraps up this year’s series of contributions on fossils. Over the year, we have looked at the fascinating world of fossils from folklore and culture to trade, complexities of ownership, considerations for collection, preparation and preservation of fossils, evolutionary traits from LUCA to LUCY, apex marine predators of the Jurassic and the many ways in which fossils help decode the subsurface. Whatever your interest in fossils, I am sure you will agree that they offer a fascinating insight into the world beneath our feet and the history of our planet!