During oil and gas exploration programmes, companies accept there will be failures (such as dry holes or low-rate wells), and use the portfolio effect to ensure that overall, the value of discovered volumes exceed the program costs.
However, this is not acceptable in SCS projects. Failure to inject the contracted CO2 volumes or contain CO2 in the target reservoir could result in project shutdown. At the extreme, there is potential for environmental or material damage, or loss of life.
Therefore, a shift is required from focussing on geological success that is common practice in hydrocarbon exploration programmes, to a broader assessment of chance of failure (risk) throughout the life of a project.
Learnings from past projects
To help ensure all potential risks are assessed, past projects should be carefully studied, particularly those that failed or experienced unexpected events. While subsurface carbon storage is relatively new, the process of storing injected fluids underground is not. Therefore, the history of these operations provides insights into the types and frequency of unexpected events.
Underground fluid storage projects have an impressive track record, but they also have a history of more than two hundred failure events worldwide (Evans, 2009, Schultz and Evans, 2020). Whilst most failures are associated with leakage up old wellbores or at surface facilities, geological factors are responsible for a small but significant fraction of injection project failures:
1) A lower-than-expected seal capacity of the overlying caprock,
2) The migration of injected fluids along fractures or across faults previously undetected or considered sealing,
3) An overestimate of the volume available for storage,
4) The migration of fluids away from the injector in an unanticipated direction, and
5) Induced seismicity.
Incidents in the case of methane injection or water injection include:
• Castor Project, Spain: Gas injection into a depleted gas field caused failure along a previously undetected fault below the reservoir and induced seismicity within 3 days of commencing injection, leading to project shutdown (Vilarrasa et al., 2021)
• Oklahoma, US: Oilfield-produced water injected into deep wells has been causing seismicity along basement faults.
• Long Beach, California: Water injected into an oil field aquifer for pressure support moved downdip, pressuring-up wells in an adjacent oil field (Allen, 1977).
Despite a shorter history, examples exist of CO2 injection projects that encountered unexpected events, which in some cases led to project shutdown, and demonstrate the relevance of a good monitoring program:
• In Salah, Algeria: CO2 injected into the water leg of a gas field was expected to migrate towards the structural closure to the west, but instead migrated through fault zones to the north, causing the entry of CO2 into a legacy borehole and fracturing of the seal (White et al., 2014).
• Snøhvit, Norway: Storage capacity of the Tubåen reservoir fell short of expectations due to sub-seismic faults and reservoir heterogeneities which caused the reservoir pressure to quickly increase to the calculated fracture pressure (Hansen et al., 2013).
• Gorgon, Australia: Sand influx into water re-injection wells has resulted in a shortfall in injected volumes. In 2021, 2.26 Mt CO2 was injected, compared to planned capacity of 4 Mt (Robertson and Mousavian, 2022).
An understanding of what caused these events provides important lessons that should be applied when planning for future geological carbon storage projects.
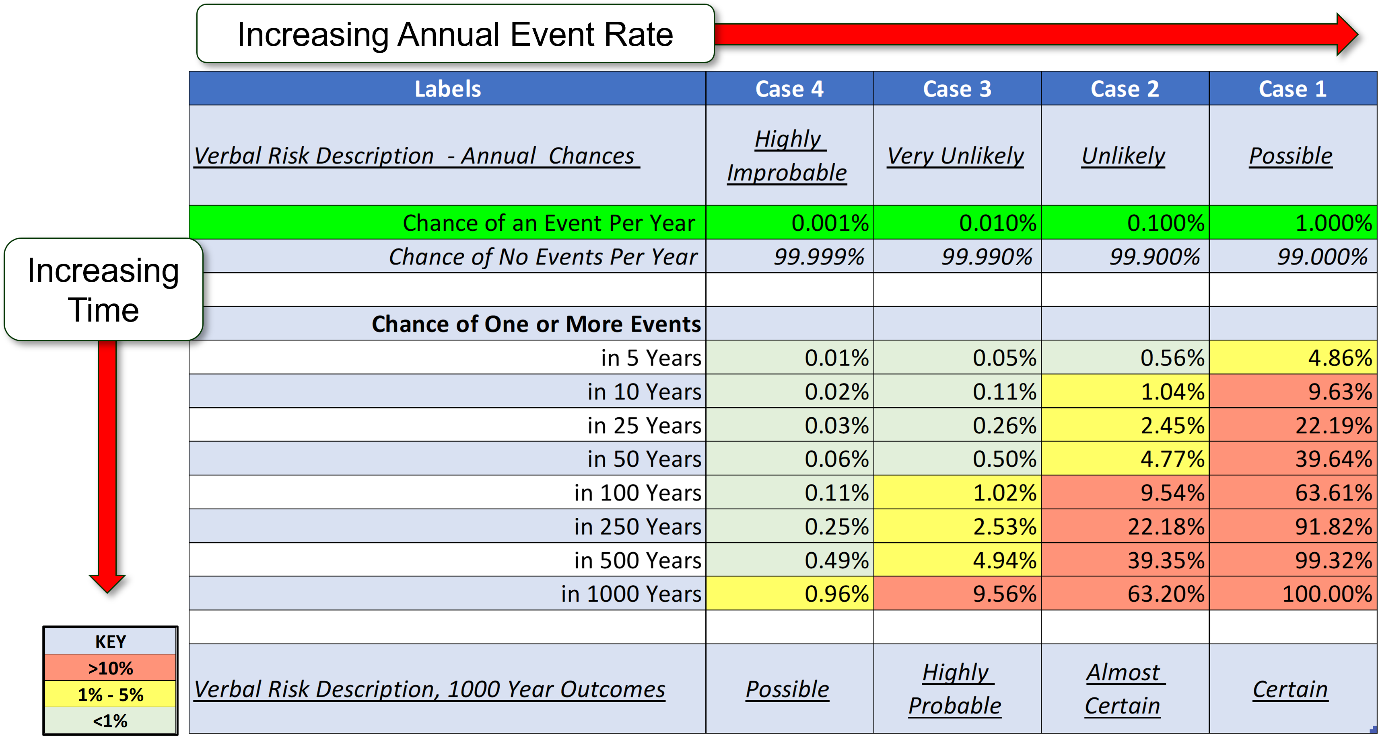
Adapting oil and gas risk assessments to carbon storage projects
Assessing the risk in an SCS project does require an assessment of geological success similar to that of evaluating a hydrocarbon prospect. However, there are several key differences and adaptations required for a thorough risk assessment:
1) Risk assessments must incorporate long timeframes,
2) The frequencies of events are likely to be very low, in the range of 1% per year and below, far lower than usual exploration chance assessments,
3) We cannot use expert judgment to differentiate the chance of an event occurring at such low frequencies, and therefore need a way to consistently assign risks to low-frequency events.
To address these challenges, we recommend using frequency data from industry databases and published sources. Where historical frequency data is unavailable, a qualitative verbal risking scale, linked to an expanded log scale of probabilities appropriate to represent low-frequency events can be applied.
An example of such a linked qualitative and quantitative risk scale was presented by Watson (2014) and is utilised in the table above. While imperfect in absolute terms, if such a scheme is applied consistently, the relative risk of events throughout the project life can be assessed.
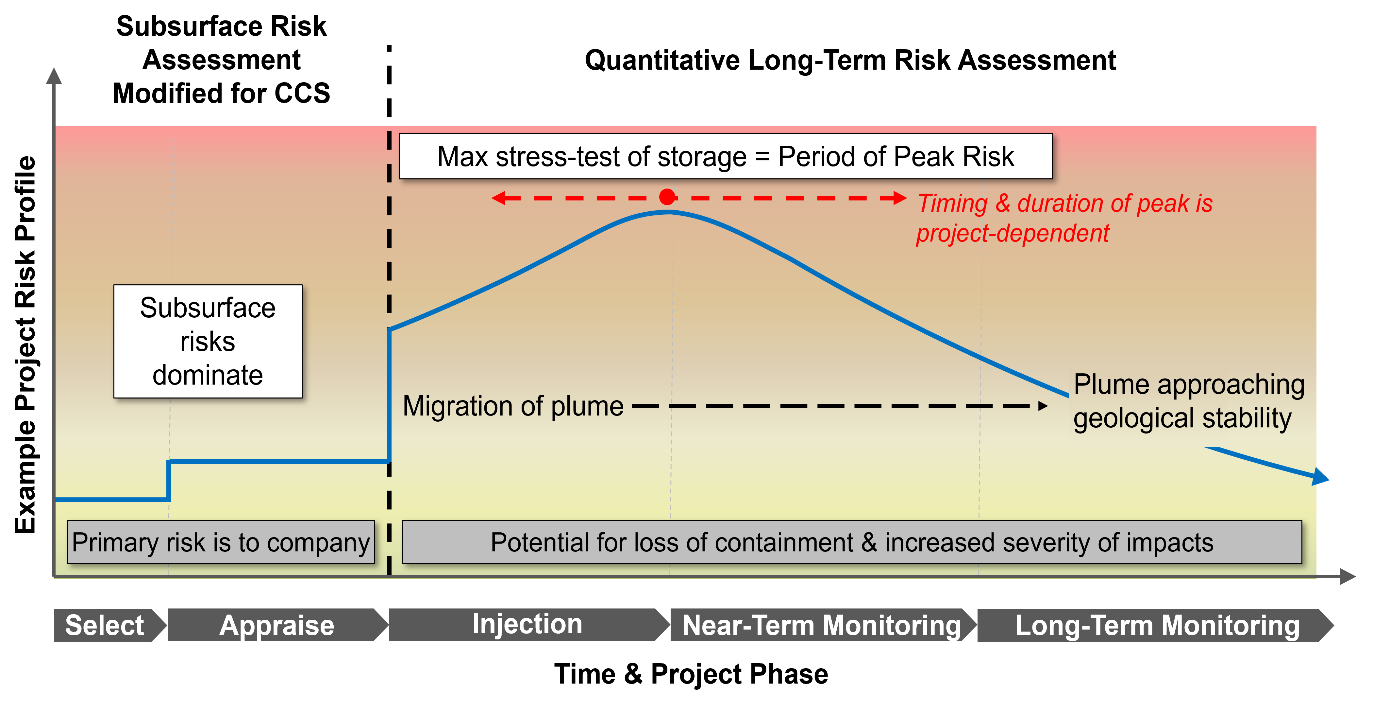
The impact of time on risk assessments
The cumulative impact of long timeframes on risk over the full lifecycle of a project is not generally appreciated. As a simple demonstration of this, the table shows four Monte Carlo scenarios run over timeframes of 5 to 1,000 years at different annual event frequency rates of between 0.001% and 1% per year. These results show that an event with an annual frequency of 0.1% is “unlikely” to occur on an annual basis, however over 1,000 years there is a 63% chance, or “almost certain” chance, of one or more events occurring.
In reality, the overall risk profile of an event will change over the life of the SCS project. During the injection phase all risks are stress tested. Project risk is then expected to decline gradually during the post-injection phase as the CO2 plume stabilises. This general model leads to the concept of “Peak Risk” for a project when the storage container is put under maximum stress, a concept also referred to by Espie and Woods (2014) and demonstrated here.
Low tolerance
Any unexpected event occurring during the lifetime of an SCS project could have consequences beyond the companies directly involved in the project. The tolerance for failure in SCS projects from the public and regulators will likely be low. Companies must demonstrate a clear understanding of the risks of a project, the impacts that events can have, and how those risks will be mitigated to obtain and maintain their Social License to Operate. It is therefore important to use a robust and thorough quantitative risk assessment workflow that addresses the full lifecycle of a project.
C. JENKINS, P. PESTMAN, P. CARRAGHER, Rose and Associates
R.M. CONSTABLE, Constable Energy Consulting Ltd.
References
Allen, D.R., 1977, Summary Report “Fo” Sand over Hydrostatic Pressure Area, North Flank, Long Beach Unit (unpublished).
Espie, T. and Woods, A. (2014). Testing some common concepts in CO2 storage. Energy Procedia 63, 5450-5460.
Evans, D.J. (2009). A review of underground fuel storage events and putting risk into perspective with other areas of the energy supply chain. In Evans, D. J. & Chadwick, R. A. (eds) Underground Gas Storage: Worldwide Experiences and Future Development in the UK and Europe. The Geological Society, London, Special Publications, 313, 173–216.
Hansen, O., Gilding, D., Nazarian, B., Osdal, B., Ringrose, Ph., Kristoffersena, J.-B., Eiken, O. and Hansen, H. (2013). Snøhvit: The history of injecting and storing 1 Mt CO2 in the fluvial Tubåen Fm. Energy Procedia 37, 3565-3573.
Robertson, B. and Mousavian, M. (2022). Gorgon Carbon Capture and Storage: The Sting in the Tail. Institute for Energy Economics and Financial Analysis. https://ieefa.org/wp-content/uploads/2022/03/Gorgon-Carbon-Capture-and-Storage_The-Sting-in-the-Tail_April-2022.pdf. Accessed 30/06/2022.
Schultz, R. A. and D. J. Evans (2020). “Occurrence frequencies and uncertainties for US underground natural gas storage facilities by state.” Journal of Natural Gas Science and Engineering 84: 103630.
Vilarrasa, V., De Simone, S., Carrera, J., & Villaseñor, A. (2021). Unraveling the causes of the seismicity induced by underground gas storage at Castor, Spain. Geophysical Research Letters, 48, e2020GL092038.
Watson, M. (2014). Containment risk assessment. In: Cook, P. (ed). Geologically storing carbon: Learning from the Otway Project experience, CSIRO publishing, 129-140.
White, J.A., Chiaramonte, L., Ezzedine, S., Foxall, W., Hao, Y., Ramirez, A. and McNab, W. (2014). Geomechanical behavior of the reservoir and caprock system at the In Salah CO2 storage project. PNAS, 111, 8747–8752.